Word Gems
exploring self-realization, sacred personhood, and full humanity
Quantum Mechanics
Dr. Fritjof Capra
Before Dirac, matter had been viewed as elementary units, indestructible and unchangeable, or as composite objects to be broken up into constituent parts; again and again, until finally arriving at some smallest indivisible units. But, after Dirac’s discovery, it was learned that, when two particles collide, they break into pieces, but these are not smaller than the original particles. The new particles are of the same kind. We can divide matter again and again, but we never obtain smaller pieces. The particles are thus destructible and indestructible at the same time.
|
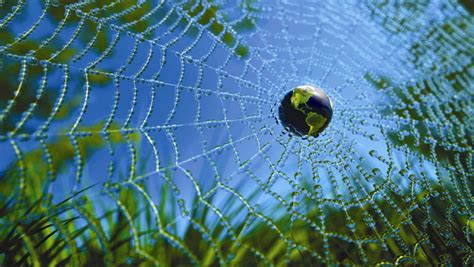
return to "Quantum Mechanics" main-page
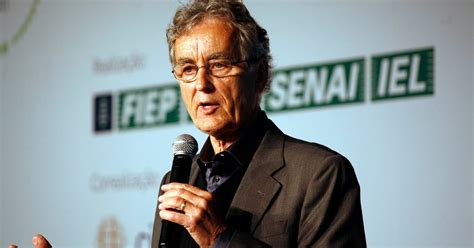
Fritjof Capra received his Ph.D. in theoretical physics from the University of Vienna in 1966 and spent 20 years doing research in theoretical high-energy physics, including at the University of Paris, the University of California at Santa Cruz, the Stanford Linear Accelerator Center, Imperial College, University of London, and the Lawrence Berkeley Laboratory at the University of California. He also taught at the University of California, Santa Cruz; the University of California, Berkeley; and San Francisco State University.
Capra has been the focus of more than 60 television interviews, documentaries, and talk shows in Europe, the United States, Brazil, Argentina, and Japan, and has been featured in major newspapers and magazines internationally. He was the first subject of the BBC’s documentary series, Beautiful Minds.
|
website: https://www.fritjofcapra.net/
Excerpts from chapter four of Dr. Fritjof's book, The Tao Of Physics
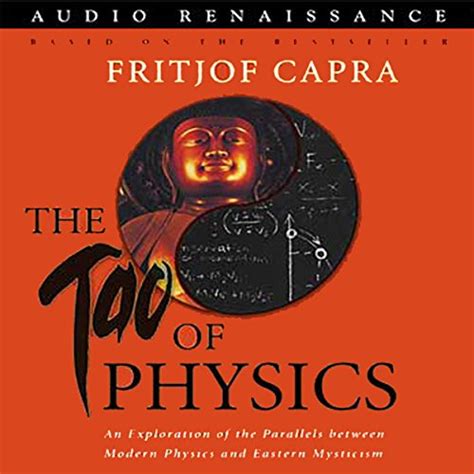
The concepts of quantum theory were not easy to accept even after their mathematical formulation had been completed. Their effect on the physicists’ imaginations was truly shattering.
Rutherford’s experiments had shown that atoms, instead of being hard and indestructible, consisted of vast regions of space in which extremely small particles moved, and now quantum theory made it clear that even these particles were nothing like the solid objects of classical physics.
The subatomic units of matter are very abstract entities which have a dual aspect. Depending on how we look at them, they appear sometimes as particles, sometimes as waves; and this dual nature is also exhibited by light which can take the form of electromagnetic waves or of particles...
The whole development started when Max Planck discovered that the energy of heat radiation is not emitted continuously, but appears in the form of ‘energy packets’. Einstein called these energy packets ‘quanta’ and recognized them as a fundamental aspect of nature. He was bold enough to postulate that light and every other form of electromagnetic radiation can appear not only as electromagnetic waves, but also in the form of these quanta.
The light quanta, which gave quantum theory its name, have since been accepted as bona fide particles and are now called photons. They are particles of a special kind, however, massless and always travelling with the speed of light.
The apparent contradiction between the particle and the wave picture was solved in a completely unexpected way which called in question the very foundation of the mechanistic world view - the concept of the reality of matter. At the subatomic level, matter does not exist with certainty at definite places, but rather shows ‘tendencies to exist’, and atomic events do not occur with certainty at definite times and in definite ways, but rather show ‘tendencies to occur’.
In the formalism of quantum theory, these tendencies are expressed as probabilities and are associated with mathematical quantities which take the form of waves. ... They are not ‘real’ three-dimensional waves like sound or water waves. They are ‘probability waves’, abstract mathematical quantities with all the characteristic properties of waves which are related to the probabilities of finding the particles at particular points in space and at particular times.
All the laws of atomic physics are expressed in terms of these probabilities. We can never predict an atomic event with certainty; we can only say how likely it is to happen. Quantum theory has thus demolished the classical concepts of solid objects and of strictly deterministic laws of nature. At the subatomic level, the solid material objects of classical physics dissolve into wave-like patterns of probabilities, and these patterns, ultimately, do not represent probabilities of things, but rather probabilities of interconnections.
A careful analysis of the process of observation in atomic physics has shown that the subatomic particles have no meaning as isolated entities, but can only be understood as interconnections between the preparation of an experiment and the subsequent measurement.
Quantum theory thus reveals a basic oneness of the universe. It shows that we cannot decompose the world into independently existing smallest units.
As we penetrate into matter, nature does not show us any isolated ‘basic building blocks’, but rather appears as a complicated web of relations between the various parts of the whole. These relations always include the observer in an essential way. The human observer constitutes the final link in the chain of observational processes, and the properties of any atomic object can only be understood in terms of the object’s interaction with the observer.
This means that the classical ideal of an objective description of nature is no longer valid. The Cartesian partition between the I and the world, between the observer and the observed, cannot be made when dealing with atomic matter. In atomic physics, we can never speak about nature without, at the same time, speaking about ourselves.
The new atomic theory could immediately solve several puzzles which had arisen in connection with the structure of atoms and could not be explained by Rutherford’s planetary model. First of all, Rutherford’s experiments had shown that the atoms making up solid matter consist almost entirely of empty space, as far as the distribution of mass is concerned.
But if all the objects around us, and we ourselves, consist mostly of empty space, why can’t we walk through closed doors? In other words, what is it that gives matter its solid aspect?
A second puzzle was the extraordinary mechanical stability of atoms. In the air, for example, atoms collide millions of times every second and yet go back to their original form after each collision. No planetary system following the laws of classical mechanics would ever come out of these collisions unaltered.
But an oxygen atom will always retain its characteristic configuration of electrons, no matter how often it collides with other atoms. This configuration, furthermore, is exactly the same in all atoms of a given kind. Two iron atoms, and consequently two pieces of pure iron, are completely identical, no matter where they come from or how they have been treated in the past.
Quantum theory has shown that all these astonishing properties of atoms arise from the wave nature of their electrons…
The atomic orbits ... are very different from those of the planets in the solar system, the difference arising from the wave nature of the electrons. An atom cannot be pictured as a small planetary system. Rather than particles circling around the nucleus, we have to imagine probability waves arranged in different orbits. Whenever we make a measurement, we will find the electrons somewhere in these orbits, but we cannot say that they are ‘going around the nucleus’ in the sense of classical mechanics.
In the orbits, the electron waves have to be arranged in such a way that ‘their ends meet’, i.e. that they form patterns known as ‘standing waves’. These patterns appear whenever waves are confined to a finite region, like the waves in a vibrating guitar string… It is well known from these examples that standing waves can assume only a limited number of well-defined shapes. In the case of the electron waves inside an atom, this means that they can exist only in certain atomic orbits with definite diameters.
The electron of a hydrogen atom, for example, can only exist in a certain first, second or third orbit, etc., and nowhere in between. Under normal conditions, it will always be in its lowest orbit, called the ‘ground state’ of the atom. From there, the electron can jump to higher orbits if it receives the necessary amount of energy, and then the atom is said to be in an ‘excited state’ from which it will go back to its ground state after a while, the electron giving off the surplus energy in the form of a quantum of electromagnetic radiation, or photon.
The states of an atom, i.e. the shapes and mutual distances of its electron orbits, are exactly the same for all atoms with the same number of electrons. This is why any two oxygen atoms, for example, standing-wave patterns in a vibrating string will be completely identical. They may be in different excited states, perhaps due to collisions with other atoms in the air, but after a while they will invariably return to exactly the same ground state. The wave nature of the electrons accounts thus for the identity of atoms and for their great mechanical stability.
A further characteristic feature of atomic states is the fact that they can be completely specified by a set of integral numbers, called ‘quantum numbers’, which indicate the location and shape of the electron orbits. The first quantum number is the number of the orbit and determines the energy an electron must have to be in that orbit; two more numbers specify the detailed shape of the electron wave in the orbit and are related to the speed and orientation of the electron’s rotation.
The fact that these details are expressed by integral numbers means that the electron cannot change its rotation continuously, but can only jump from one value to another, just as it can only jump from one orbit to another. Again the higher values represent excited states of the atom, the ground state being the one where all the electrons are in the lowest possible orbits and have the smallest possible amounts of rotation.
Tendencies to exist, particles reacting to confinement with motion, atoms switching suddenly from one ‘quantum state’ to another, and an essential interconnectedness of all phenomena - these are some of the unusual features of the atomic world. The basic force, on the other hand, which gives rise to all atomic phenomena is familiar and can be experienced in the macroscopic world. It is the force of electric attraction between the positively charged atomic nucleus and the negatively charged electrons.
The interplay of this force with the electron waves gives rise to the tremendous variety of structures and phenomena in our environment. It is responsible for all chemical reactions, and for the formation of molecules, that is, of aggregates of several atoms bound to each other by mutual attraction. The interaction between electrons and atomic nuclei is thus the basis of all solids, liquids and gases, and also of all living organisms and of the biological processes associated with them.
In this immensely rich world of atomic phenomena, the nuclei play the role of extremely small, stable centres which constitute the source of the electric force and form the skeletons of the great variety of molecular structures. To understand these structures, and most of the natural phenomena around us, it is not necessary to know more about the nuclei than their charge and their mass. In order to understand the nature of matter, however, to know what matter is ultimately made of, one has to study the atomic nuclei which contain practically all of its mass.
Author’s footnote: The ‘rotation’ of an electron in its orbit must not be understood in the classical sense; it is determined by the shape of the electron wave in terms of the probabilities for the particle’s existence in certain parts of the orbit.
In the 1930s, after quantum theory had unravelled the world of atoms, it was therefore the main task of physicists to understand the structure of nuclei, their constituents and the forces which hold them together so tightly.
The first important step towards an understanding of nuclear structure was the discovery of the neutron as the second constituent of the nucleus, a particle which has roughly the same mass as the proton (the first nuclear constituent) – about two thousand times the mass of the electron - but does not carry an electric charge.
This discovery not only explained how the nuclei of all chemical elements were built up from protons and neutrons, but also revealed that the nuclear force, which kept these particles so tightly bound within the nucleus, was a completely new phenomenon. It could not be of electromagnetic origin since the neutrons were electrically neutral. Physicists soon realized that they were here confronted with a new force of nature which does not manifest itself anywhere outside the nucleus.
An atomic nucleus is about one hundred thousand times smaller than the whole atom and yet it contains almost all of the atom’s mass. This means that matter inside the nucleus must be extremely dense compared to the forms of matter we are used to. Indeed, if the whole human body were compressed to nuclear density it would not take up more space than a pinhead.
This high density, however, is not the only unusual property of nuclear matter. Being of the same quantum nature as electrons, the ‘nucleons’ - as the protons and neutrons are often called - respond to their confinement with high velocities, and since they are squeezed into a much smaller volume their reaction is all the more violent. They race about in the nucleus with velocities of about 40,000 miles per second!
Nuclear matter is thus a form of matter entirely different from anything we experience ‘up here’ in our macroscopic environment. We can, perhaps, picture it best as tiny drops of an extremely dense liquid which is boiling and bubbling most fiercely.
The essential new aspect of nuclear matter which accounts for all its unusual properties is the strong nuclear force, and the feature that makes this force so unique is its extremely short range. It acts only when the nucleons come very near to each other, that is, when their distance is about two to three times their diameter. At such a distance, the nuclear force is strongly attractive, but when the distance becomes less the force becomes strongly repulsive so that the nucleons cannot approach each other any closer. In this way, the nuclear force keeps the nucleus in an extremely stable, though extremely dynamic equilibrium.
The picture of matter which emerges from the study of atoms and nuclei shows that most of it is concentrated in tiny drops separated by huge distances. In the vast space between the massive and fiercely boiling nuclear drops move the electrons. These constitute only a tiny fraction of the total mass, but give matter its solid aspect and provide the links necessary to build up the molecular structures. They are also involved in the chemical reactions and are responsible for the chemical properties of matter.
Nuclear reactions, on the other hand, generally do not occur naturally in this form of matter because the available energies are not high enough to disturb the nuclear equilibrium. This form of matter, however, with its multitude of shapes and textures and its complicated molecular architecture, can exist only under very special conditions, when the temperature is not too high, so that the molecules do not jiggle too much.
When the thermal energy increases about a hundredfold, as it does in most stars, all atomic and molecular structures are destroyed. Most of the matter in the universe exists, in fact, in a state which is very different from the one just described. In the centre of the stars exist large accumulations of nuclear matter, and nuclear processes which occur only very rarely on earth predominate there. They are essential for the great variety of stellar phenomena observed in astronomy, most of which arise from a combination of nuclear and gravitational effects.
For our planet, the nuclear processes in the centre of the Sun are of particular importance because they furnish the energy which sustains our terrestrial environment. It has been one of the great triumphs of modern physics to discover that the constant energy flow from the Sun, our vital link with the world of the very large, is a result of nuclear reactions, of phenomena in the world of the infinitely small.
In the history of man’s penetration into this submicroscopic world, a stage was reached in the early 1930s when scientists thought they had now finally discovered the ‘basic building blocks’ of matter. It was known that all matter consisted of atoms and that all atoms consisted of protons, neutrons and electrons. These so-called ‘elementary particles’ were seen as the ultimate indestructible units of matter: atoms in the Democritean sense. Although quantum theory implies, as mentioned previously, that we cannot decompose the world into independently existing smallest units, this was not generally perceived at that time. The classical habits of thought were still so persistent that most physicists tried to understand matter in terms of its ‘basic building blocks’, and this trend of thought is, in fact, quite strong even today.
Two further developments in modern physics have shown, however, that the notion of elementary particles as the primary units of matter has to be abandoned. One of these developments was experimental, the other theoretical, and both began in the 1930s. On the experimental side, new particles were discovered as physicists refined their experimental techniques and developed ingenious new devices for particle detection.
Thus the number of particles increased from three to six by 1935, then to eighteen by 1955, and today we know over two hundred ‘elementary’ particles. The … adjective ‘elementary’ is no longer very attractive in such a situation. As more and more particles were discovered over the years, it became clear that not all of them could be called ‘elementary’, and today there is a widespread belief among physicists that none of them deserves this name.
This belief is enforced by the theoretical developments which paralleled the discovery of an ever-increasing number of particles. Soon after the formulation of quantum theory, it became clear that a complete theory of nuclear phenomena must not only be a quantum theory, but must also incorporate relativity theory. This is because the particles confined to dimensions of the size of nuclei often move so fast that their speed comes close to the speed of light. This fact is crucial for the description of their behaviour, because every description of natural phenomena involving velocities close to the speed of light has to take relativity theory into account. It has to be, as we say, a ‘relativist/c’ description.
What we need, therefore, for a full understanding of the nuclear world is a theory which incorporates both quantum theory and relativity theory. Such a theory has not yet been found, and therefore we have as yet been unable to formulate a complete theory of the nucleus.
Although we know quite a lot about nuclear structure and about the interactions between nuclear particles, we do not yet understand the nature and complicated form of the nuclear force on a fundamental level. There is no complete theory of the nuclear particle world comparable to quantum theory for the atomic world. We do have several ‘quantum-relativistic’ models which describe some aspects of the world of particles very well, but the fusion of quantum and relativity theory into a complete theory of the particle world is still the central problem and great challenge of modern fundamental physics.
Relativity theory has had a profound influence on our picture of matter by forcing us to modify our concept of a particle in an essential way. In classical physics, the mass of an object had always been associated with an indestructible material substance, with some ‘stuff’ of which all things were thought to be made. Relativity theory showed that mass has nothing to do with any substance, but is a form of energy. Energy, however, is a dynamic quantity associated with activity, or with processes. The fact that the mass of a particle is equivalent to a certain amount of energy means that the particle can no longer be seen as a static object, but has to be conceived as a dynamic pattern, a process involving the energy which manifests itself as the particle’s mass.
Dirac's work helped us to see that particles are affected by the 'special theory of relativity'
This new view of particles was initiated by Dirac when he formulated a relativistic equation describing the behaviour of electrons. Dirac’s theory was not only extremely successful in accounting for the fine details of atomic structure, but also revealed a fundamental symmetry between matter and anti-matter. It predicted the existence of an anti-electron with the same mass as the electron but with an opposite charge. This positively charged particle, now called the positron, was indeed discovered two years after Dirac had predicted it.
The symmetry between matter and antimatter implies that for every particle there exists an antiparticle with equal mass and opposite charge. Pairs of particles and antiparticles can be created if enough energy is available and can be made to turn into pure energy in the reverse process of annihilation.
These processes of particle creation and annihilation had been predicted from Dirac’s theory before they were actually discovered in nature, and since then they have been observed millions of times.
The creation of material particles from pure energy is certainly the most spectacular effect of relativity theory, and it can only be understood in terms of the view of particles outlined above. Before relativistic particle physics, the constituents of matter had always been considered as being either elementary units which were indestructible and unchangeable, or as composite objects which could be broken up into their constituent parts; and the basic question was whether one could divide matter again and again, or whether one would finally arrive at some smallest indivisible units.
After Dirac’s discovery, the whole question of the division of matter appeared in a new light. When two particles collide with high energies, they generally break into pieces, but these pieces are not smaller than the original particles. They are again particles of the same kind and are created out of the energy of motion (‘kinetic energy’) involved in the collision process. The whole problem of dividing matter is thus resolved in an unexpected sense.
The only way to divide subatomic particles further is to bang them together in collision processes involving high energies. This way, we can divide matter again and again, but we never obtain smaller pieces because we just create particles out of the energy involved in the process. The subatomic particles are thus destructible and indestructible at the same time.
This state of affairs is bound to remain paradoxical as long as we adopt the static view of composite ‘objects’ consisting of ‘basic building blocks’. Only when the dynamic, relativistic view is adopted does the paradox disappear.
Editor's note: We've seen this assertion before. To cling to the metaparadigm that matter consists of "hard little billiard balls" and is fundamental to reality is to invite paradox and confusion.
The particles are then seen as dynamic patterns, or processes, which involve a certain amount of energy appearing to us as their mass. In a collision process, the energy of the two colliding particles is redistributed to form a new pattern, and if it has been increased by a sufficient amount of kinetic energy, this new pattern may involve additional particles...
The collisions of particles are our main experimental method to study their properties and interactions, and the beautiful lines, spirals and curves traced by the particles in bubble chambers are thus of paramount importance for modern physics.
The high-energy scattering experiments of the past decades have shown us the dynamic and ever-changing nature of the particle world in the most striking way. Matter has appeared in these experiments as completely mutable. All particles can be transmuted into other particles; they can be created from energy and can vanish into energy. In this world, classical concepts like ‘elementary particle’, ‘material substance’ or ‘isolated object’, have lost their meaning; the whole universe appears as a dynamic web of inseparable energy patterns...
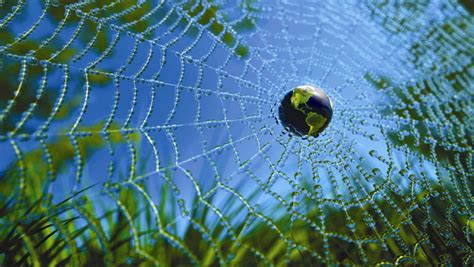
The fact that particles interact through forces which manifest themselves as the exchange of other particles is yet another reason why the subatomic world cannot be decomposed into constituent parts. From the macroscopic level down to the nuclear level, the forces which hold things together are relatively weak and it is a good approximation to say that things consist of constituent parts. Thus a grain of salt can be said to consist of salt molecules, the salt molecules of two kinds of atoms, those atoms to consist of nuclei and electrons, and the nuclei of protons and neutrons.
At the particle level, however, it is no longer possible to see things that way. In recent years, there has been an increasing amount of evidence that the protons and neutrons, too, are composite objects; but the forces holding them together are so strong or - what amounts to the same - the velocities acquired by the components are so high, that the relativistic picture has to be applied, where the forces are also particles. Thus the distinction between the constituent particles and the particles making up the binding forces becomes blurred and the approximation of an object consisting of constituent parts breaks down.
The particle world cannot be decomposed into elementary components. In modern physics, the universe is thus experienced as a dynamic, inseparable whole which always includes the observer in an essential way. In this experience, the traditional [Newtonian] concepts of space and time, of isolated objects, and of cause and effect, lose their meaning.
Such an experience, however, is very similar to that of the Eastern mystics. The similarity becomes apparent in quantum and relativity theory, and becomes even stronger in the ‘quantum-relativistic’ models of subatomic physics where both these theories combine to produce the most striking parallels to Eastern mysticism.
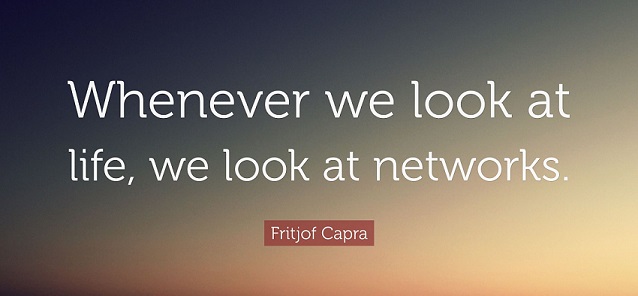
'a continual popping into existence from the void'
from Dr. Jim Al-Khalili:
Einstein balked at the notion of uncertainty at the core of reality. A crisis developed in physics with two seemingly opposing branches, Relativity and Uncertainty.
How could these two disparate views of reality be married?
English physicist Paul Dirac in 1928 began to bridge the divide. His famous “Dirac Equation” is sometimes called “the most beautiful equation in the world.”
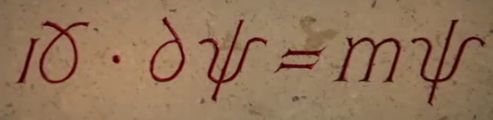
Dirac’s mathematics predicted a continual virtual popping into existence, from the seeming void, matter and anti-matter particles, most of which would almost instantly annihilate each other. However, one in a billion of these particles would not be destroyed but remain. These survivors become the matter of the universe, the material stuff of which all things consist.
.jpg)
quotations by Paul Dirac
“The measure of greatness in a scientific idea is the extent to which it stimulates thought and opens up new lines of research.”
“Living is worthwhile if one can contribute in some small way to this endless chain of progress.”
“When you ask what are electrons and protons I ought to answer that this question is not a profitable one to ask and does not really have a meaning. The important thing about electrons and protons is not what they are but how they behave, how they move. I can describe the situation by comparing it to the game of chess. In chess, we have various chessmen, kings, knights, pawns and so on. If you ask what chessman is, the answer would be that it is a piece of wood, or a piece of ivory, or perhaps just a sign written on paper, or anything whatever. It does not matter. Each chessman has a characteristic way of moving and this is all that matters about it. The whole game of chess follows from this way of moving the various chessmen.”
“People who equate all the different kinds of human activity to money are taking too primitive a view of things.”
“If one is working from the point of view of getting beauty into one's equation, ... one is on a sure line of progress.”
“In science one tries to tell people, in such a way as to be understood by everyone, something that no one ever knew before. But in poetry, it's the exact opposite.”
“Pick a flower on Earth and you move the farthest star.”
“The successful development of science requires a proper balance to be maintained between the method of building up from observations and the method of deducing by pure reasoning from speculative assumptions.”
“If you are receptive and humble, mathematics will lead you by the hand.”
“God is a mathematician of a very high order and He used advanced mathematics in constructing the universe.”
“Mathematics is only a tool and one should learn to hold the physical ideas in one's mind without reference to the mathematical form.”
“The mathematician plays a game in which he himself invents the rules while the physicist plays a game in which the rules are provided by nature, but as time goes on it becomes increasingly evident that the rules which the mathematician finds interesting are the same as those which nature has chosen.”
“It seems clear that the present quantum mechanics is not in its final form. Some further changes will be needed, just about as drastic as the changes made in passing from Bohr's orbit theory to quantum mechanics. Someday a new quantum mechanics, a relativistic one, will be discovered, in which we will not have these infinities occurring at all. It might very well be that the new quantum mechanics will have determinism in the way that Einstein wanted.”
Editor’s note: It is, for me, more than a small matter of interest, Dirac’s views on beauty in science, the limitations of mathematics (and this, by one of history’s foremost mathematicians), and how Einstein might yet be proven correct in his determinism. I’d come to the same conclusions; especially noteworthy is Dirac’s sense that, despite what appears to be a profligate randomness at the heart of quantum mechanics, if we could view things from a higher mountain, Einstein’s sense of determinism might yet be shown to be the overarching, controlling precept of the cosmos.
|
What is commonly viewed as “nothing,” or space, or the void, is a hot-bed of creative activity. Virtual particles, “quantum fluctuations” in the void, come into existence, spring from the matrix of hidden quantum fields of probability.
The “emptiness” and the “nothingness” of space contain the deepest mysteries and secrets of the cosmos.
|